Beyond Schrödinger’s cat: Quantum effects in biology
The weirdness of quantum physics is changing our understanding of biology
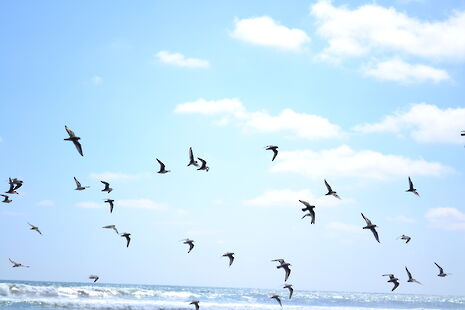
Quantum mechanics is not just the spooky, headline-making phenomena of spontaneous teleportation, or intercontinental particle entanglement. At its most basic, it is stated that energy is quantised, and at the minute scales where that is significant, it causes non-classical behaviour – that is to say, it can’t be explained by classical physical models. It is this element of chance that scared Einstein, who proclaimed that “God does not play dice with the universe.” Quantum biology looks for such behaviours in biological systems, applying well-validated physics to a novel area. Quantum physics has shown the existence of these non-classical processes, and if utilising such phenomena conferred a fitness advantage to living organisms, then there is no biological reason why evolution would not have ended up using them.
Meanwhile, physicists become sceptical because observations of these processes within physics typically must be carried out in an extremely well-controlled environment, often near absolute zero. The idea that they play a role in “warm, wet, and noisy” biological scenarios seems unlikely to many at first. However, successes in the field so far appear to rally against this.
There have been successful intersections of quantum biology with a wide variety of biological disciplines, from protein biochemistry to animal behaviour. For example, there is now strong evidence that plants rely on quantum energy transfer to gather together the energy from sunlight to start photosynthesis. Quantised energy moves as excitons (think of them as particles) through the light-harvesting complex along multiple paths at once, resulting in almost 100% efficient transfer. Sceptical biologists: there’s a good reason for evolutionary selection. And for sceptical physicists: there is evidence that vibrational coupling of proteins helps maintain this despite the abundance of environmental noise (to put it simply).
In animals, an explanation for the mechanism of the sense of smell has been proposed, involving electron tunnelling. In this case, each odorant has a specific vibrational energy and, when interacting with a receptor, this can allow electron tunnelling across the receptor. However, the most notable success has been in understanding a different sense: magnetoreception.
Many birds can navigate according to the Earth’s magnetic field, and it now seems that this depends on quantum effects. The “radical pair mechanism” essentially relies on spin quantisation for unpaired electrons on radical species. It proposes that the transitions between different discrete spin states are affected by the orientation of an applied magnetic field relative to the radicals’ own, and that the different states lead to alternative chemical reactions. Thus, magnetic field direction can change the ratios of radical spin states and thus affect chemical reaction products, which can be used as a magnetoreceptor in a biochemical system. The radical pairs are initially produced by photoexcitation of cryptochrome proteins, and for this to be viable the radicals’ movement must be restricted relative to the external magnetic field. There is growing evidence that cryptochrome proteins are capable of this as well.
With these contributions and others besides, quantum biology has slowly but surely developed since the mid-20th century and is now improving our understanding of what have previously been mysterious biological phenomena. What it proposes would surely surprise whoever first asked the questions of how birds navigate the skies, or how solar energy transferred to plants
Features / 3am in Cambridge
25 June 2025Comment / Why shouldn’t we share our libraries with A-level students?
25 June 2025News / Revealed: Emmanuel relies most on postgrad supervisors
28 June 2025News / Gardies faces dissolution
27 June 2025Sport / Sport, spectacle, and sanctioned collisions: May Bumps 2025
25 June 2025