The magic of mRNA
mRNA vaccines are just the tip of the iceberg. Ruby Jackson discusses how this versatile molecule is revolutionising medicine
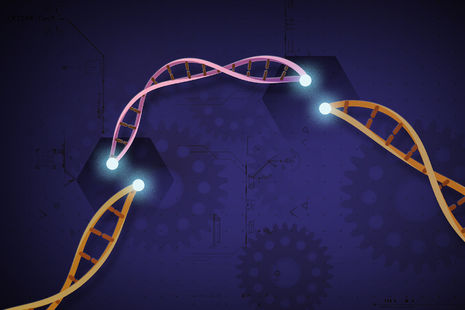
mRNA has been spending a lot of time in the spotlight lately. Three years ago it shot to fame for its role in the Pfizer-BioNTech and Moderna vaccines against Covid-19. In the last month, it’s made the headlines again, this time as part of a vaccine for treating lung cancer. In fact, these are just two of a myriad of ways this versatile molecule, central to the function of all living cells, is transforming medicine. mRNA technology is a powerful tool that could be used to treat many previously devastating genetic diseases, reprogram the process of cellular ageing, and even edit our genes.
“mRNA technology could be used to treat many previously devastating genetic diseases”
Ribonucleic acid, or RNA, is a molecule related to DNA, although with a slightly different structure. There are many forms of RNA, each with a particular shape and set of roles within the cell. The role of messenger RNA, or mRNA, is information transfer: the information encoded in DNA is copied into a molecule of mRNA in a process known as transcription. This mRNA copy then enters the cytoplasm (the watery main compartment of the cell), while the precious DNA remains inside the nucleus, protected from damage.
Once in the cytoplasm, this mRNA molecule acts as a template that is used by the cell’s protein-making machinery. The particular protein that is built from a strand of mRNA depends on the structure, or sequence, of that particular mRNA molecule; mRNAs with different sequences encode the instructions for different proteins.
This role of mRNA, providing a kind of molecular recipe for protein synthesis, is exploited by mRNA-based medical treatments. By causing cells to take up mRNA of a particular sequence, their protein-making machinery can be employed to produce the corresponding protein. Using this model, any cell can be stimulated to produce any protein, provided it is treated with the appropriate mRNA. This incredible versatility means mRNA technology can be adapted to generate a huge range of treatments.
Vaccines
The mRNA in vaccines is taken up by dendritic cells, a type of immune cell that act as first responders to foreign substances in the body. In the Covid vaccines, the particular mRNA used contains instructions for the viral spike protein, while cancer vaccines contain mRNA that causes dendritic cells to make tumour-specific antigens (TSAs): specific proteins that are found on tumour cells, but not healthy cells.
The dendritic cells then synthesise these proteins (whether spike or TSA) and present them to T cells, the soldiers of the immune system, teaching the T cells to look out for these proteins. This presentation effectively primes the immune system - if these T cells encounter these antigens in future, either on particles of virus during an infection, or on the cells of a cancerous tumour, they can more quickly recognise and destroy these cells.
Protein replacement therapy
Many genetic diseases result from cells not making enough of a particular protein, or producing proteins that are the wrong shape. Haemophilia A is caused by a genetic mutation that means cells in the liver do not produce enough factor VIII. This protein is vital for the formation of blood clots, meaning individuals with this condition are prone to uncontrollable and life-threatening bleeds unless they receive regular infusions of factor VIII.
mRNA therapy could be used to stimulate these patients’ cells to make their own factor VIII instead. Trials in mice have already shown promise, and if the technology were adapted for use in humans, it could reduce the frequency that people need to receive treatments (from once every 48 hours or so to weekly), as well as lowering costs.
Using mRNA to give cells back their ability to make the correct proteins shows potential for treating other diseases too, including cystic fibrosis and diabetes.
Stem cell therapeutics
While most cells in the body have a shape and structure that is adapted to their particular functions (for example, muscle cells contain lots of mitochondria to supply energy for contraction, and red blood cells are packed with haemoglobin for oxygen transport), stem cells are unspecialised. A stem cell is like a blank slate - it has the capacity to divide and become specialised to produce cells of any of a range of different types.
Stem cells have incredible potential for repairing damaged tissues, as they can be guided into differentiating into a particular type of cell, providing a reservoir of new cells to replace the damaged ones. The difficulty is that although adults have some stem cells, these are limited in the number of different cell types they can become. Some stem cell therapies use stem cells taken from embryos or umbilical cords, which are more versatile. However, obtaining these cells comes with its own set of logistical and ethical challenges that means it will likely never be possible to adapt it for wide-scale use. Enter mRNA.
Some research has managed to use mRNA to cause adult cells to make particular proteins, called transcription factors, which then trigger the adult cells to revert back to their unspecialised stem-cell state. The cells produced this way are called induced pluripotent stem cells (iPSCs), and can be transplanted into different tissues to help with regeneration. Heart muscle and nerve tissue are notoriously limited in their capacity for regeneration, and so this therapy shows huge potential for treating the damage caused by heart attacks or injuries to the brain and spinal cord.
Gene editing
It’s impossible to mention mRNA without a nod to its roles in gene editing. The CRISPR-Cas9 gene editing system, which won the Nobel Prize in Chemistry in 2020, is named after the repeating sections of bacterial DNA, known as clustered regularly interspaced short palindromic repeats, from which it is produced.
“It’s impossible to mention mRNA without a nod to its roles in gene editing”
Bacteria use this molecular system to cut the DNA of viruses attempting to attack the bacterial cells, but it can be adapted to alter human DNA. The mRNA used in CRISPR acts as a guide that directs the Cas9 (which stands for CRISPR-associated) enzyme to a particular section of DNA. Here the enzyme acts like a pair of molecular scissors and cuts the DNA, enabling sections of DNA to be removed or inserted at a particular position, depending on the sequence of the mRNA used.
A lot more research will be required before CRISPR-Cas9 can be widely used in human patients, but editing DNA in this precise way offers the potential to cure diseases ranging from sickle cell anaemia to cancer.
News / Dropouts at Cambridge fall to five-year low
9 June 2025Features / The privilege of passion: is “following your dreams” a status symbol?
8 June 2025News / Robinson students sent elsewhere to wash clothes
10 June 2025Music / We are so back: Stereolab and the politics of feeling again
10 June 2025News / News in Brief: TikTok, confessions pages, and a mystery for the ages
8 June 2025