Saving bananas from extinction
In this issue of Cambridge Spotlight, Hayoung Choi explores work at Cambridge focussing on grafting techniques at the equivalent embryonic stage of plant development and how such techniques can solve some of our agricultural problems
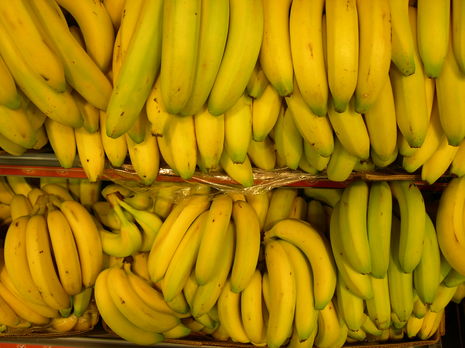
Biting into the white, soft flesh of banana, you may easily notice that a banana has no seeds. That may not be surprising, since that was always how a banana was to you, but for bananas, no seeds mean an existential risk – which is not the first one they have faced in their seedless history. The bananas we eat are triploids (3n), meaning that they have three sets of chromosomes instead of two like most organisms (including humans). With more genetic material, triploids grow faster and larger, leaving humans abundant flesh to feed on, but they are infertile. They are unable to arrange their three sets of chromosomes into pairs, which is necessary for the successful generation of gametes, or sex cells, by the process of meiosis.
“With more genetic material, triploids grow faster and larger, leaving humans abundant flesh to feed on, but they are infertile"
As a result, bananas have to be asexually propagated by a bulb or rhizome, pieces of the mother plant bearing the same genetic code. The banana you had three months ago is basically the same organism as the banana you have now. So, a disease fatal to any individual banana has the potential to become a disastrous pandemic. Gros Michel, the previous prevalent commercial banana variety, faced the end of their era as the uncureable Panama disease (first reported in 1876) spread to all banana-producing regions by the 1950s. Even though the Cavendish variety succeeded Gros Michel, our banana supply is still at risk as Cavendish is susceptible to another fungal disease, Black Sigatoka. It’s as if the agricultural horticulture industry is in an endless race to develop a big, delicious banana variety that will survive the next few decades.
Developing novel crops is not a valid goal in banana species alone. The agricultural industry spends much time, money, and effort in developing fruits and vegetables that are convenient to eat, have better fragrance and taste, higher fertility, and the ability to grow in barren soil, or have pest- and disease-resistance. An especially useful technique from ancient times is grafting, the act of putting together portions of different plant species. If it was animal tissues that were joined together – by means such as organ transplants – there would be a rapid immune response of attacking the non-self-tissue. However, plants have an immune system largely based on chemicals – the presence and activity of microbial proteins are detected, but non-self-plant tissues are off the radar. Plants rather focus on healing the wound. As water flows through the vascular system now merged at the graft union, chemicals and plant hormones will be shared, turning the plant into a physiological hybrid that bears chimaeric fruits. Therefore, grafting is a straightforward way to add a characteristic of one plant to another.
The key process for successful grafting is the tissue regeneration that links two plants. This is done by meristematic tissue, which is why grafting should be done at the interface of meristematic tissues. These are tissues comparable to animal stem cells in that they possess the potential to differentiate into diverse cell types. Especially, the ‘vascular cambium’ in stems is responsible for regeneration of the xylem and phloem – passages for water and nutrients. However, cambium is only present in dicotyledons (dicots), but absent in monocotyledons (monocots). In dicots, different tissue layers develop as concentric rings; the vascular cambium is between xylem and phloem tissue layers, producing new vascular cells and secondarily thickening the stem. In contrast, monocots develop several bundles of xylem and phloem and lack meristematic tissue; they are incapable of secondary thickening of the stem as well as the traditional way of grafting. To date, horticulturists have developed various dicots through grafting, but no monocots. The grafting potential of monocots lay dormant, despite them encompassing the most agriculturally significant plants, including cereal grains, wheats, grasses, and bananas.
The Monocot Side of Grafting
In the recent study, a Cambridge research team discovered a successful method of grafting monocots. This monocot grafting method is different to other plant grafts, which are usually done on fully grown plants, in that it is done at the embryonic stage where the more complex features of the plant are yet to manifest. It is the first time for monocots that grafting has been tried at the embryonic stage, but the method is common among animals – you might have come across news on human-animal chimaera embryos and accompanied ethical controversies. An early-stage animal embryo consists of stem cells with broad potential. However, after a certain developmental stage, the destiny of embryonic cells is settled, so that you can identify which area will turn into the head and which will be the tail. The same is true with the plant cell, and in a monocot embryo, it is an area called the ‘hypocotyl’ that becomes the boundary between the to-be-root and to-be-shoot cells. The researchers grafted monocots at the hypocotyl. By grafting plants before the shoot and roots started to form, researchers achieved monocot hybridisation comparable to traditional grafting of meristematic tissue.
The success of the novel grafting method was proven through a number of experiments. The researchers observed cells shorten in the fused area and the movement of fluorescent dyes through both xylem and phloem. These results show that the cells at the interface were dividing to merge the two portions and connecting the vascular system; the grafted plant is ready for its new state of life. Additionally, the resultant plant chimera successfully reached maturity.
Once monocots are grafted, researchers studied what made the grafting successful: the molecular mechanisms of healing wounds. When they studied gene expression patterns in grafted monocots, they noticed that similar genes were participating in merging the two plants. A more interesting bit of the story is that through the comparison between monocot and dicot gene expression patterns, the researchers identified some dicot genes that had not previously been connected with the tissue regeneration.
The Potential of Plants
Other than the meristematic tissue healing and merging the cut site, the key to understanding plant development is plasticity. That is, plants can develop different numbers of branches and leaves in different places – which is in contrast to how animals develop arms, legs, and internal organs in more or less predestined numbers and locations. The plasticity is closely linked to limited mobility: while keeping their body rooted to the soil, plants can branch out and grow leaves in the direction of sunlight. Plasticity implies less specific developmental stages as an embryo, greater tolerance of developmental errors, and from there arises an indirect evolutionary advantage in the long term – plants readily go through hybridisation and polyploidy, which is their major evolutionary driving force. The most significant example is the one that brought us the everyday meal. During the Neolithic era, a wheat crop hybridised with a tough and weedy goat grass – and brought about the bread wheat Triticum aestivum, tolerant to cold, pests, and diseases.
Will scientists be able to create a new crop as significant as wheat? That will take years of searching for the right formula for grafting, but for sure the novel monocot grafting method has opened a new window. It may be too early to say, but with accelerated development of crop varieties, bananas may be crossed off the endangered species list!
News / Meta opens £12 million lab in Cambridge
11 July 2025Lifestyle / Reflections on rowing
10 July 2025News / Write for Varsity this Michaelmas
13 July 2025Features / How to catch a coat thief
13 July 2025Comment / What is originality, anyway?
14 July 2025