Science research: picks of the year
Varsity selects the best of Cambridge’s research papers from the three main disciplines
Biology: stem cells and development
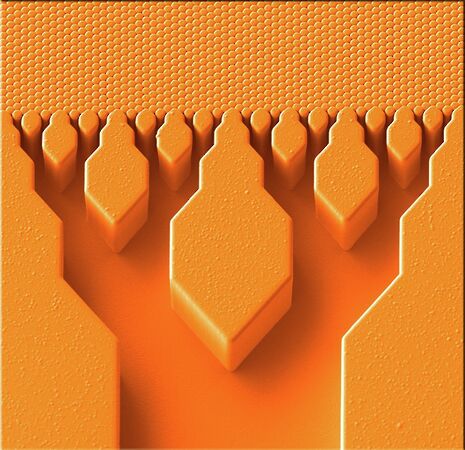
Shudong Li
The University of Cambridge has always been at the forefront of development and stem cell research. Supporting the university in this rapidly changing field are two internationally renowned centres – the Stem Cell Institute and the Gurdon Institute. Researchers focus on stem cells in particular cells due to their incredible ability to alter themselves to become any type of cell in the human body. A single stem cell has the theoretical potential to regenerate and heal damaged organs, making its clinical uses near endless.
This academic year, there have been plenty of new findings involving the use of human stem cells. For the first time, so-called ‘naïve’ pluripotent stem cells from humans have been derived from early embryos. The isolated cells have the most potential to change and are almost completely flexible.
At a different research group in the university, strong evidence has discovered that human pluripotent stem cells will in fact develop normally when added to an embryo. This has significant implications for regenerative medicine, silencing critics that cite the unknown dangers of erroneous development.
It was also in only May this year that research from Professor Zernicka-Goetz’s group hit the headlines. Her team were able to keep human embryos alive and developing outside of the body for an incredible 13 days after fertilisation. In doing so they were able to gain a deeper understanding of just how humans change and alter during the earliest stages of development. New techniques like this and the knowledge gained by using them will help make procedures like IVF more efficient and safe – an example of transition towards a clinical setting.
Physics: new use for nanopores
Sam Watcham
The ability to accurately detect and identify individual constituents of a biological sample containing many different molecules (such as proteins or DNA) is an expanding area of interdisciplinary research, encompassing elements of both physics and biochemistry. If a reliable method of protein identification can be found, the potential diagnostic applications will be enormous.
One attractive possibility is to use ‘nanopores’ – nanometre-sized holes in a membrane that typically only allow a single molecule through at a time – for this purpose. The idea is simple: the membrane is placed in salty water, and an ionic current is driven across the membrane using an applied voltage. If a protein or DNA molecule passes through a nanopore, it will block part of the current. The effect of this will be dependent on the shape, charge and structure of the molecule, which can then be inferred.
In theory this technique could allow all the different molecules within a given sample to be uniquely identified. Up until now, however, this has not been realised in practice.
In February, researchers Nicholas Bell and Ulrich Keyser at the Cavendish Laboratory published a paper in which they used the technique of DNA origami (whereby intricate 3D structures can be built by folding DNA) to create a library of nanometre-sized DNA structures. By driving these structures through custom solid-state nanopores, they were able to identify which structure was passing through a nanopore with 94 per cent accuracy. This is a large improvement over other techniques, many of which can only target one molecule at a time.
In addition, the authors went on to functionalise four of their structures to detect a single, specific antibody (a protein that targets foreign cells such as viruses). The four antibodies were then simultaneously detected from a sample, the first time this has been achieved using nanopore sensing. The results open up exciting possibilities for the detection of molecules associated with specific diseases, which will allow their structures to be investigated.
Chemistry: an ‘electric revolution’
Jon wall
Over the past 10 years, automotive experts have been promising an “electric revolution” in response to predicted shortages of oil as well as ongoing climate change. Each time their predictions have been largely stymied by one factor: a lack of range on electric cars. Such cars are useful in cities, but cannot cross whole countries.
As such, development of better batteries is a key research area. In Cambridge, this is led by Professor Clare Grey at the Department of Chemistry. In a paper from this year’s Michaelmas term, her team announced its discovery of a new design for lithium-air batteries which promises high energy efficiency.
Lithium-air cells achieve as close to the limits for energy density in a battery as is possible, and so are seen as having significantly greater potential than conventional lithium-ion batteries. However, these lithium-air batteries face substantial problems with the conditions under which they can operate – until now, carbon dioxide, nitrogen and water all had to be removed from the environment for the cells to work.
However, Professor Grey’s team have developed a new system in which the cell can tolerate water being present, while maintaining high energy efficiency and good rechargeability. This new design introduces lithium iodide as an additive, which changes the key reaction of the cell. In standard lithium-air cells, Li2O2 is formed and reformed, whereas in the new design LiOH is used instead.
However, there are still some issues to be resolved: there are still constraints on the reaction conditions, and the prototypes take days, rather than minutes, to charge and discharge. While a commercial battery is around a decade away, this research offers a new way of creating better lithium-air batteries, and, when these stronger batteries do become available, the “electric revolution” in motoring may finally occur.
News / Academics lead campaign against Lord Browne Chancellor bid
2 July 2025News / Clare students call on College to divest
3 July 2025Lifestyle / It’s pretty fun to talk to strangers
3 July 2025Science / It’s only rocket science, Elon
3 July 2025News / Join Varsity‘s editorial team this Michaelmas
23 June 2025